These New Oceans
– – Sir Arthur C. Clarke
Chapter 1
The Spheres of Titan
No one seems to believe, here at the dawn of incomparable promise and peril, that we are the luckiest generation – and potentially the most tragic – to have tread upon this Earth. But for the here and now, let’s talk about luck, you and I. Let’s talk about the fantastic. Let’s talk about how, though difficult to believe, our view of the stars and the planets has been shifting every day – shifting like wax – from the barren and the familiar and the commonplace, to the utterly alien.
With submersibles and deep-ocean robots, we have probed a hidden world where fields of undersea geysers protrude through the bed of the Atlantic like the spires of a lost city – and all around and beneath the volcanic spires, the world is a feeding frenzy, curiously slow and stately, and in every direction, animals no one has ever seen before. The latest generation of robots penetrate jets of vent-water emerging four times hotter than steam, but the overlying miles of ocean press down with such force as to forbid the water from boiling, though it emerges hot enough to glow… with such light as to provide deep-ocean beacons for swarms of Van Dover shrimp. In such places, life itself must have had one of its many starts.
The vent-penetrating robot, outside our view-port, is impossible to behold, down here, without the mind wandering up from the sea floor to the planets and the stars beyond. How can we not wander, from the seas to space, knowing that these very same “bots” are the direct ancestors to the machines we will be hurling toward icebound seas circling Jupiter, Saturn, and beyond? How can we not wander, and let our imaginations race, when our work down here is really a dry run for “up there”?
Looking quickly around our own solar system, we see five probable candidates for subsurface seas and lakes (Mars; Jupiter’s Europa and Ganymede; Saturn’s Enceladus and Titan), and two possible locations (Jupiter’s Callisto; Neptune’s Triton). One Sun system, seven chances for subsurface seas – and only one chance for a world with just the right mass to hold onto its hydrogen (and hence water), if it sustained just the right amount of geologic activity and were placed just the right distance from just the right kind of star. Looking outward and outward, it becomes possible to believe that most life in the universe exists near deep-ocean volcanic vents hidden under ice, inside worlds that turn out to have no need of atmospheres in order to cook up life, nor even a need for the right kind of star. Anyplace warm and wet and underground will serve. And it becomes possible to believe, too, that worlds with their habitable zones on the outside are so rare as to make we surface dwellers a galactic minority, if not downright freakish.
In our constantly shifting view of our own tiny solar system, we should not really be viewing Titan as a mere moon of Saturn, and Saturn as a planet-proper. Titan, with an atmosphere denser than Earth’s, and with a diameter exceeding the planet Mercury’s, is more Earth-like than Mars or Venus and would surely have been called a planet were it flung freely and on its own course, away from Saturn. The “planet” Saturn appears to be equally misnamed. Saturn and Jupiter are “gas-giant planets” – with the “gas giant” phrase deserving more emphasis. Indeed, were Saturn able to hold its shape and to be placed on the surface of a huge ocean with a gravitational field only equal to Earth’s, the gas-giant “planet” would actually float. Probably, there have been few things in heaven and on Earth more misnamed, mistreated, and misunderstood than Saturn and Jupiter (save perhaps for Stephen Jay Gould’s Irish elk – which was neither Irish nor an elk). Rather than being called giant planets, they should more accurately be called dwarf stars. Most stars within view of our telescopes are parts of binaries, trinaries, or even more complex stellar families. Down here on Earth, we do not appear to lie very far outside (indeed, not outside at all) of this probability curve. The asteroid belt that stretches between Mars and Jupiter is a ring around the Sun, analogous in every way to the rings around Saturn and Jupiter. Likewise, Jupiter and Saturn are analogous in every way to solar systems orbiting within our solar system – each with its own set of orbiting planets. They differ from our central star merely in not having accumulated enough mass to burn brightly as second or third suns in our sky. They dwell somewhere near the lower limit of what we would call brown dwarf stars, or “cold stars.”
Of the worlds orbiting these not-fully-formed stars (sometimes called “failed,” or dead stars), four of them – Europa and Ganymede, the second and third moons of Jupiter; and Enceladus and Titan, the second and sixth moons of Saturn – are quite alive, at least in the geologic sense.
Tugged alternately this way by their cold stars, and that way by their sister satellites, some of these ice worlds, creaking and snapping and groaning throughout, are kept warm and wet inside by tidal friction. These are worlds whose icy crusts are girdled by continent-sized grooves and whose molten lava is melted ice. From the time robotic camera sleds first revealed these worlds close up (beginning with the Voyager space-probes of the early 1980s), scientists and engineers began concocting schemes to verify the existence of those seas of molten ice, and to descend into them. Plans have ranged from robot helicopters for Titan to robot submarines and (in one particularly fanciful proposal) an actual spaceship submarine under the ice of Europa, crewed by both human and dolphin astronauts.
Judging from what we have seen so far in our solar system, the average ocean-bearing ice-world has at its center a rocky core roughly the size of our moon. The surface of this core is actually the bottom of a sea hundreds of kilometers deep and enclosed in a protective shell of slush and ice up to 820 kilometers (500 miles) thick. We already know from studying meteorites, and from spectroscopic analyses of eruptions on Jupiter’s moon Io, and from the sands of Mars, that sulfur is ubiquitous in the solar system, and that the floors of icebound seas inside Titan or Ganymede are probably laced with an ample supply of sulfides. We also know, from submersible and robotic exploration of Earth’s own ocean floor that as long as sulfides are available to make food, entire ecosystems can flourish in worlds without suns.
One of the smallest, and oddest hidden seas, probably circles Saturn inside Enceladus. It is possible that cracks in the moon’s surface penetrate through to its sea and act as vents. Since Enceladus has no atmosphere, water rising through these vents would erupt as a gas rather than as a liquid. Because surface temperatures never exceed a noontime high of minus 180 degrees Centigrade (-293 deg. F) on Enceladus, erupted water, as it flashes to vapor and jets into space, would crystalize so quickly that many of the flakes must inevitably fall back to the surface – which perhaps explains why Enceladus’ surface reflects about as much sunlight as does fresh-fallen snow. Some of the needles and flakes would escape Enceladus’ unusually low gravity, rising more than a hundred kilometers above the ground, unfailingly escaping Enceladan orbit and forming a snowstorm in space. This, together with the fact that the densest part of Saturn’s outermost (albeit thinnest) ring, coincides with Enceladus’ orbit (implicating Enceladus as the source of the ring), points to the probable existence of an underground ocean.
During the early morning hours of July 1, 2004, the Cassini probe descended below Enceladus and toward a breaking maneuver into Saturnian orbit. At approximately twenty-five kilometers per second, it plunged through trillions of twinkling ice crystals and dust motes, most of them (according to impacts recorded against Cassini’s sensors) smaller than the individual molecules of soot contained in cigarette smoke. Careful observation of the moonlet’s surface, taken together with its mass and its motion within Saturn’s gravitational field, and within the fields of Enceladus’ sister satellites, hinted at a center of once-molten glass and iron (with a faint possibility that the core might still be at least marginally volcanic). Its outer core would thus be lighter material of carbonaceous meteorite composition, having long ago floated to the surface like a silt-stone froth tossed upon liquid iron – with still-lighter water and ice floating above the silt-stone. Unlike carbonaceous meteorites (or their parent, asteroidal bodies), Enceladus had not frozen all the way through to its center, some four billion years ago. If biology can be summed up as the production of order from chaos, then evolution, the process of chaos with feedback, might never have died down there, in the depths of Enceladus – for she is a world caught almost forever in a gravitational tug-of-war. On one side, no farther away than Earth is from the moon, but a hundred times more massive than Earth, is Saturn. On the other side are Dione (a 1,150 kilometer, or 700 mile-wide satellite that approaches within one half the distance separating Earth from the moon) and Tethys (a 1,082 kilometer, or 660 mile-wide moon that sweeps past Enceladus at a hair-raising, near-collision radius only twice as large as Earth’s circumference, or 1/5th the distance between the Earth and the moon. For billions of years, the alternating gravitational jolts have been dumping enormous loads of frictional heat into Enceladus’ interior; and despite the fact that the world is small enough (at a width of only 500 kilometers) to fit comfortably within the eastern and western borders of Kansas, it is one of the most geologically lively, and strangest, bodies in the solar system.
Enceladus has a density of 1.1 grams per cubic centimeter; only ten percent greater than if the worldlet had been built out of pure water-ice. Adding to this oddity, an unrelenting gravitational flexing, reflected in a twisted and grooved surface with curious extrusions of ice as soft as toothpaste, and reflected too in a surface albedo of 100% (consistent with purest white snow), the existence of a new ocean to be explored becomes a physical and mathematical certainty. However, this new ocean can enclose a rocky core no more than 156 kilometers (95 miles) across. The core – the actual surface of Enceladus – is literally a world within a world, a planet so small that a submersible or a robot, driving forward at only 20.5 kilometers (12.5 miles) per hour, could travel all the way around its 492 kilometer (300 mile) circumference in a single day and night of exploration. A world within a world… overlain by an ocean as many as a hundred kilometers deep, overlain by an equally thick, and protective, self-sealing mantle – or shell – of slush and ice.
Because of its combination of small size and low gravity, temperatures and pressures in Enceladus’ underground sea may closely parallel those found on Earth’s abyssal plains. Current estimates of the rate of tidal heating inside Enceladus dictate that a sea more than a hundred kilometers thick, overlain by soft ice reaching a hundred kilometers higher to the world’s surface, cannot possibly be sustained by a volcanic core only 95 kilometers wide – if indeed the world within Enceladus is still volcanically active at all. Soft ice and internal water, therefore, must have a melting point at least slightly lower than pure water-ice. The fluid is likely mixed with salts, methane clathrates, and/or hydrates of ammonia (much as water in Earth’s oceans is mixed with salt, and has a lower melting point than plain, freshwater). Numbers can be dull and boring and seemingly irrelevant – especially when encountered in the sorts of problems typically assigned in a school-boy’s homework (or, to quote MTV’s Beavis: “I hate numbers; there’s – like – too many of them). It’s a pity that more school systems don’t make more interesting play out of numbers – as in, applying them to puzzling out the possibilities of life in an Enceladan sea. The big secret about science (which we scientists have been very weak at communicating) is in a word that most people rarely associate with ice and volcanic rock and numbers. The numbers behind – or beneath – Enceladus are… fun. They tickle our in-born love of the mysterious; they are the beginning of a fantastic adventure. We are probably not alone in this universe. And we may have neighbors much nearer than most people believe – right here in our own Solar System. The numbers tell us so.
A further playing with and puzzling out of numbers, of surface areas and volumes against rates of heat loss and heat input – of pressures exerted by overlaying kilometers of ice and rock – had allowed some of us to make good guesses about one of the worlds next door to Enceladus, as far back as 1981. It came as no surprise, therefore, when Cassini revealed, with cloud-piercing instruments, a world amazingly like Earth, with mountain ranges and continental rifting, and signs of alluvial plains and even glaciers – which, like the earth’s active and “young” surface, had scabbed over and healed most of its asteroid impact scars. The numbers suggest (the rules of geometry and gravitation alone suggest) that if you dig down deep enough into Titan, you will probably find water. But to reach it, either you or a geologic fissure-turned-volcanic vent would have to penetrate so many miles of crust and mantle that the sheer pressure of overlaying mass produces different pressure phases, or densities, of ice. These layers are real-life variations of Kurt Vonnegut’s superdense “Ice Nine,” a fictional substance in his novel, Cat’s Cradle. The greater the pressure under which ice is formed, the more closely packed are the molecules of water and the lower the ice’s melting point (at least, initially). We know of at least ten solid forms of water, from Ice One (ordinary ice cubes) to Ice Ten, suspected to exist inside Neptune.
Approximately 102 kilometers (62 miles) below Titan’s surface, you should begin to encounter a layer of Ice Three. It melts at minus twenty degrees Centigrade (- 5 deg. F), and with each deepening kilometer, the melting point falls deeper still. If one atmosphere is the measure of sea level air pressure on Earth, we encounter Ice Three at a pressure of 2,200 atmospheres. For comparison, 150 atmospheres is the pressure inside an early 21st century power plant boiler… 200 atmospheres is the pressure at which sea-water on Earth will implode a fully-charged scuba tank… 600 atmospheres is the threshold at which a mosquito’s snout needles down through human skin (which is more than sufficient, if applied simultaneously to millions of points over its entire surface, to crush a fully-charged scuba tank)… 800 atmospheres is the peak pressure of a fist against a skull during a karate strike… 900 atmospheres is a zone of pressure comparable to the greatest depth of an earthly ocean. Thus, at 2,200 atmospheres, Ice Three forms at a pressure 2.44 times higher than the bottom of Earth’s Mariana Trench (11 kilometers, or 6.7 miles deep). Deeper still, the melting point of Ice Three continues to plunge toward minus 34.7 degrees centigrade, and it may plunge a little beyond that if (as is probably the case) salts, ammonia, crystalline forms of methane, and other non-water substances are present.
Though far below freezing, minus 40 degrees Centigrade is both figuratively and literally hot lava by the standards of the outer solar system. Below Titan’s crust – and sometimes sending up eruptions more than a hundred kilometers through it – the Ice Three layer must resemble an unstable labyrinth of faulting and blocking amid watery magma pools. Were you able to descend, as in Jules Verne’s Journey to the Center of the Earth, through a volcanic pipe in Titan’s surface, your attempts to understand how a magma chamber can be kept “warm” and fluid at this distance from the sun must include sub-surface methane, all around you. At the surface, Titan’s atmosphere is slightly more than two percent methane (CH4). Ultraviolet radiation from the sun presently converts methane, photochemically, into ethane (C2H6) and propane (C3H8), at a rate that should have removed virtually all of this methane from the atmosphere in “only” a few million years. While ethane and propane are in fact present in abundance, the present-day atmospheric concentration of methane should not be there, on a world more than 4 billion years old, unless, within the past few million years, and probably therefore into time present, Titan’s surface was being recharged by cryo-volcanic surges from the interior. More than a hundred kilometers down, in the Ice Three labyrinth, the water-methane ocean would conduct to you the sounds of the world’s surface quivering and snapping, as if the planetary shell were trying continually to pull itself apart, straining to break into great crustal blocks. As the world, in a near-gravitational lock, rotating at only 100 meters per second with one side always facing Saturn, bobs in and out of Saturn’s magnetic field (at a distance averaging three times the gap between the earth and the moon), the Ice Three sea and the solid crust of Titan must bob up and down too, lifting mountains and crustal plates and their hydrocarbon stream-beds several feet, producing an otherworldly tide must put the Bay of Funday to shame. Probably, the greater part of Titan’s unstable, Ice Three sea – and perhaps all of it – exists on the Saturn-facing side, much as the magma seas beneath Earth’s moon (which bled out, about 3.7 billion years ago, through giant asteroid punctures, and froze to form the dark Lunar “Maria,”) formed only on the earth-facing side.
Deeper inside Titan, at a pressure of 3,500 atmospheres and a depth of more than 150 kilometers (nearly 100 miles), the melting point of high-pressure Ice Three has risen to minus 17 degrees Centigrade (freshwater in sea level air on Earth melts at Zero degrees Centigrade). Approximately 150 kilometers deeper, at 6,300 atmospheres, the melting point, were the water salt-and-methane-free, would be rising to 2 degrees Centigrade (requiring an almost comfortable 36 deg F). Below 300 kilometers, and above 10,000 atmospheres, the melting point continues rising steadily with depth, through boundaries of Ice Four and Ice Five “granite” and “slush,” and below that, a more stable, inner planetary shell of Ice Six. There, the ice melts at a searing seventy-five degrees Centigrade (only 25 deg. C below the boiling point of water). There may be enough heat, however, beneath the Ice Six shell, to produce yet a second sub-surface sea above the world’s solid rock surface.
Titan’s radius, from the center to the surface, is 2575 kilometers (1570 miles); and careful observation of Cassini’s gravitational interaction with Titan gave an accurate determination of Titan’s mass and density (1.95), suggesting a rock-to-ice ratio of 52:48. This means that the layers of ice and water reach rock bottom at a depth of about 920 kilometers (or 561 miles). The stony interior of Titan – the world within the world – would thus have a diameter of about 3310 kilometers (2018 miles). With an equatorial radius approximately equal to the Earth’s moon, with a thick insulation of water and ice and with at least moderate gravitational “dumping” from Saturn’s tidal bulge (which nudges Titan’s velocity ever so slightly upward and moves it outward, as Saturn simultaneously slows its rotation, ever so slightly), might have sustained and stabilized volcanic activity of Titan’s rock-interior far beyond the mere billion year, geologically active life of Earth’s moon. The heat of formation and the same accumulation of radioactive elements that produces volcanic mid-ocean ridges and pliable continental plates on Earth should also have been operating inside Titan, more than 3.5 billion years ago. But because Earth has a diameter 3.85 times that of Titan’s rock interior, and because as objects decrease in size, surface areas (relative to volume) through which internal heat can be radiated to space, shrink slower than volumes. The meaning of this is that small worlds cool faster, and to substantially greater depths, than large worlds. Earth is still active, with a relatively thin planetary skin, and with giant lakes of molten basalt lying just beneath the surface. By contrast, our moon’s entire mantle has been geologically dead for more than 3 billion years, frozen solid to a depth of more than 900 kilometers. Mars is, in every way, the world in between. Its diameter is half Earth’s, twice that of the moon, and twice that of the insulated world within Titan. It is neither dead, like the moon, nor so active that it is shrouded in volcanic seams, with entire continents adrift. Mars’ crust cooled and rigidified to greater depths than Earth’s, so that continental plates locked into place more than 3 billion years ago. Instead of the Hawaiian islands, and North America drifting away from Europe, a huge canyon opened up more than half way around the planet, and a volcano so tall that it soars nearly three times the height of the Mauna Loa volcano (from the sea floor to its mountain-top telescopes), until it pokes clear through the Martian atmosphere and into space. The true surface of Titan, insulated from space, may be more similar to Mars than to the moon, with one or more large volcanoes huddled on one hemisphere, and with the ice above, here and there still flowing liquid – and, perhaps, here and there flowing with life’s surge.
The innermost sea, beneath the Ice Six, most certainly existed during the first 2 billion years of Titan’s history. Then, like Earth and the moon, like Mars an Enceladus, the rocky mantle and metal-rich core were heated by the decay of radioactive elements (including rare and very hot, short-lived isotopes of aluminum and magnesium, producable only by supernovae explosions and stirred into the matrix of our oldest meteorites almost at the “moment of our solar system’s formation). These elements alone, working throughout the volume of inner Titan, pushed temperatures high enough to liquefy rock. Lava welled up from the interior, and the surrounding ice absorbed its heat and melted. As radioactive elements continued to decay and their abundance dwindled, internal heat inevitably began failing to keep pace with sea-floor cooling. Though once liquid (below the Ice Six layer, through the entire sea-floor expanse of Titan), since approximately 2.5 billion BC, the deep-Titan biosphere must have begun to steadily freeze, except for a few oases clustered closely around dying volcanoes. During the past 1.5 to 2 billion years, the radioactive glow might even have retreated so deep that from a sea-floor perspective it has simply gone out, and whatever life started down there may now be nothing more than fossils.
Still… even today there exists that equally strange, upper level between the Ice Six mantle and Titan’s surface, where (with or without the addition of what would promise to be a remarkably chaotic mantle convection involving multiple pressure phases of ice) – water will probably remain liquid below minus 40 degrees Centigrade. The result is an ice-world encircling sea – a bubble of water, perhaps 200 kilometers (122 miles) thick, locked between two concentric spheres of ice. Rocks, silt, and organic dust that would normally be mixed in with the ice should have settled on top of the inner sphere of Ice Six, littering that upper sea-floor with clays, sulfides, and other life-sustaining ingredients. This sea, assuming it still exists, is as old as Earth, old enough for living cells, and perhaps even for full-fledged creatures, to have evolved.
An imaginary descent from Titan’s cloud-tops through its subsurface seas to its lowermost sea-floor suggests a reference system that has no precedent, and perhaps no equal. Concepts of “air” and “rock” and “ocean” become elastic and interchangeable. Water is lava. Ice is rock. Methane gas is ice, and perhaps also the sleet that rains down from the upper reaches of Titan’s atmosphere. Here, the reality of scientific understanding catches up with and surpasses science fiction. Here, amongst seas sandwiched at different temperatures between different pressure phases of ice, one begins to see, and at least by analogy to understand, what the Buddhists must mean when they speak of multiple simultaneous planes of existence.
If (and, oh, what a great “if”) complex multi-cellular life could now or ever had existed in Titan’s seas, and if flaws or corridors in the ice also existed, life might have been able to pass up or down from one sea to another. And if one is permitted to speculate on this result – to ask, “whatever could such creatures look like?” – then (forgive me this trespass of terrestrial chauvinism), my guess is that they should appear eerily familiar, having been shaped by the same physical laws governing the forms of animals living in terrestrial oceans. Fast-swimming, torpedo-shaped, fishlike animals possessing fins and gills in just the right places come quickly to mind. Conceivably (given something akin to a sea with free oxygen, or metabolisms efficiently powered by some combination of oxygen, nitrogen, and methane), they would share the subsurface waters with other fauna reminiscent of earthly phyla, including clams, worms, and crabs. Familiar as they might seem, however, their genetic origins would belong literally to another world. A “fish” inhabiting the mantle of Titan would, in terms of ancestry, be no more related to a barracuda than a barracuda is to a Venus flytrap.
The sub-surface environment will dictate other major differences between life in its seas and life on Earth. Our own deep-sea fishes tend to spend at least portions of their lives in a sun-lit nursery ground near the surface, and at great depths they are surrounded by flashes of living light. Thus, large, light-gathering eyes are useful to them, whereas the interior of Titan or one of its companions is not likely to provide any meaningful illumination. Creatures living therein might lack eyes and color, like cave-dwelling crustaceans and fishes known here on Earth, and they would “see” their surroundings as odors and faint disturbances in the water – even as bio-electric impulses coursing through the muscles of prey and predator species.
Looking to Titan’s surface, there is yet another potential habitat for life. Titan has a rich and active atmosphere. Standing at ground level, the air pressure is 60 percent greater than at sea level on Earth. The atmosphere is mostly nitrogen. And the air is cold. Very cold indeed. At minus 180 degrees Centigrade (-293 deg. F), your hand, unprotected, would undergo a strange transformation: from the consistency of flesh to the hardness of glass. The humidity around you ranges up to 70 percent, and sometimes as high as 100 percent. It is not water humidity; it is methane humidity. Where the mountains of Titan have grown sufficiently high, they have been capped with methane snow – glaciers of methane that have flowed groundward and become rivers of methane. Methane evidently plays the same role that water plays on Earth: vapor, rain, snow, slush, and ice. Yet there is more than methane on the wind. Cassini has detected traces of ethane (H3C-CH3), acetylene (HC=-CH), propane H3C-CH2-CH3), formaldehyde (H2CO) and hydrogen cyanide (HCN). In spite of the fact that these molecules are the first chemical steps toward life, Titan’s streams and ponds appear to be far too cold to support the life-building process that could knit them into more elaborate entities such as viruses and cells. The lakes of gasoline (in time-past or in time-present) are so frigid that carbon dioxide and water – things we are used to breathing and drinking – are present everywhere as solids. They are the boulders and sand we would find in a Titanian stream. That free oxygen and carbon dioxide are absent in the atmosphere (except perhaps in the aftermath of asteroid and comet impacts) can only mean that water and “dry ice” can play no role in any imaginable near-surface biology, no role whatsoever outside of geology.
Faced with such conditions, life would appear to have no chance. But there is no reason to preclude cold, liquid hydrocarbons from playing a role similar to water in our own bacteria and cellular tissues, down here on Earth. And there is reason to believe that chemicals, even amino acids and protein-like chemicals, can become animate in hellishly cold environments. Irradiated by ultra-violet light from the Sun and bombarded by charged particles from Saturn’s magnetic field, Titan’s upper atmosphere synthesizes a broad range of organic molecules, including occasional sugars, amino acids, and other precursors to life. That methane even exists, in a charged and ultra-violet environment that would unfailingly convert it into ethane in a geologic nanosecond, speaks to some sort of volcanic replenishment of methane at the surface. Cryo-volcanism, by sheer definition, speaks of a lively energy source in Titan’s rift valleys and on volcanic peaks. The methane itself, seen gathering at high latitudes, into cloud-banks larger than Japan and France, speaks of yet another energy source. Swept by a sleet of frozen hydrocarbons, Titan’s ponds, or lakes, will accumulate organic debris – amino acids and sugars among them – from the heavens. Some of the debris will be kept afloat and concentrated by surface tension. Some will sink to the bottom and become sticky, lifeless caramel.
University of Hawaii microbiologist/astrobiologist Clair E. Folsome demonstrated (as far back as 1970) that it is easy, at least in a warm water environment, to put these compounds on the road to life. Simply apply shock waves to clumps of protein randomly assembled from amino acids, and sweetened with sugar – “you could even put them in a sealed plastic flask and let a monkey bounce them off a wall for a few minutes” – and, invariably, “sticky caramel” will self-assemble into microscopic rods and spheres and long, hollow tubes with membraneous divisions running down their lengths. They look like life, but they are not life, though they may be getting close to it. The shapes and structures we associate with cellular membranes are merely common stability forms in nature; physics dictates the shape of biology. Unfortunately, there are no monkeys with flasks on Titan, to produce the right shocks and to guarantee that the right chemistry ever gets started.
There are, however, huge methane anvil clouds, and thunderheads, and sporadic methane rainstorms, especially near Titan’s polar regions.
Most people are not aware that a major component of sea foam is naturally occurring organic fat. Only the tiniest handful of holiday visitors to Niagara Falls have ever expressed an interest in taking a closer, microscopic look at the organic froth, or “brown foam” accumulating at the feet of the falls; and even most scientists would not guess that Niagara foam could become a window on other worlds, least of all Titan. But it has, for us, become a window. Has it not?
Where there is rain, even methane rain, there must be streams and tributaries – methane rivers charting meandering courses down beds of hydrocarbon snow – there must be waterfalls or, rather, methanefalls. And where there are methanefalls, there are shock waves. And where shock waves are at work, they become next of kin to Folsome’s monkeys.
In one of the late Clair Folsome’s room-temperature experiments, when a flask of methane, ammonia and water was shaken vigorously (following several hours of amino-acid-producing electrical discharges through the mixture), more than 60 percent of the carbon present in the methane was recovered as organic microstructures – protocells – resembling clumps of 20 micron-wide spheres and tubes and smaller, bacteria-like objects.
On Titan, Cassini’s first indications of dynamic cloud patterns, and of fluid erosion near the south pole, near the place where methane condensation appeared to be strongest, suggested that the universe had already been performing the Folsome experiment on frigid methane – for many hundreds of millions of years before we were born. It rains methane and traces of liquid propane on the land; and there are streams, and methanefalls, and the question of life.
Unsolved questions lie in every direction; there are whole new worlds full of puzzles unimagined when the first human creatures set foot upon the moon. With methane streams and underground oceans, Titan has some small possibility, but a possibility nonetheless, of being able to generate life both above and below its surface. What would happen if it did, and if, through a wrinkle in the skin, liquid water should find its way to the surface? A “fish” that erupted up with the water would die violently, its blood fizzing from decompression and then freezing solid within seconds. If, before it died, the “fish” were to embrace an inhabitant of Titan’s surface, the embrace would feel like the touch of molten lead to the surface dweller. The “fish” would seem an impossible creation – deadly to the touch, living in lava, and turning to stone almost the instant it is exposed to air.
What would happen if, somewhere in the universe, a world like Titan developed intelligent life in both environments? If representatives of both were to meet and shake hands (tentacles?), one would fry and the other would freeze. If they discovered science, and learned how to make pressure suits, what kinds of contacts (or conflicts) would occur between them?
Always there are questions. Only a few of them are likely to be answered by the time Cassini/Hugens’ follow-on expeditions begin to take shape (and if we’re studying these worlds correctly, each new answer opens like a fractal doorway into a dozen new questions). And even before those follow-on expeditions can be designed, another question looms: What kind of probe do you send to an ice-world? The answer depends, in part, on which world you want to explore first. Titan is unique, but she’s not alone.
Of all the sub-surface seas begging our exploration, Jupiter’s Europa is the most accessible, though probably not the most promising, at least in terms of multicellular life. The moon next door, Io, has been too active to have any chance at all of supporting life. The entire world, from its skin down to its core, is a massive ball of lava. Europa has not fared much better. Throughout most of her existence, Europa’s sea floor has probably been too active to support life, and the world has spent the better part of 4.6 billion years venting its volatiles into space. This is why the ice at Europa’s surface is so thin that the Galileo Jupiter orbiter was able to photograph new cracks opening in the shell right before our very eyes. Such events will allow easy access to future melt-through probes, able to dispatch robot submarines into Europa’s underground seas. But Galileo also revealed that while the freshly glazed-over surface ice carried sulfur and traces of salt from below, there was no sign of free oxygen. The bed of the Europa Sea has probably known a reasonably stable existence only during the past 2 billion years, and the most advanced possible life forms down there might be bacterial mats, stromatolite colonies, and rusticle-style bacterial consortia – like Earth’s seas, about 3 billion BC, and for similar reasons.
Though Europa was always – owing to what appears to be a relatively easily breached crust – the focus of Jet Propulsion Laboratory brainstorming sessions (which reached me in New Zealand during 1981, while machines named Galileo and Cassini were only in their birth stages), contributors were encouraged to speculate freely, so long as we did not break any physical laws, and so long as we did not laugh at anyone else’s idea – “For one person’s crazy idea,” team leader Kerry T. Nock had cautioned, “might spark a thought in another person that leads to something more practical.” One session alone generated 110 hypothetical mission proposals. Amongst the more interesting plans was No. 59: dropping dolphin astronauts into Europa’s seas to search for local versions of seaweed, fish, or any other life forms – and to bring specimens back to the spaceship. Dolphins were believed ideal because they are intelligent and cooperative, and because their sophisticated sonar would enable them to locate drifting or swimming objects in the sunless sea. It’s a good idea, in theory. And in practice?
Well, there are difficulties that put the idea at least forty years beyond our present technological ability. Even before we get around to such matters arising as dolphins’ specific salinity requirements (necessitating cetacean dive suits), the animals must come up for air, and Europa is surrounded by vacuum. So, a ship of humans and “dolphinauts” would have to arrive with pressure compensation capability and moon pools (meaning an uncomfortable and unhealthy proposition called “saturation diving”). If, instead of “simply” carrying a retro-fitted Alvin or Mir deep-diving submersible to Europa, suited human and dolphin egress were part of the plan, maximum depth, to a pressure equivalent to 80 meters on Earth (barely more than .35 kilometers, or 1127 feet beneath Europa) – would be dictated by the amount of ice lying around and on top of the ship’s corridor into Europa. Additionally, the ship must get under the ice quickly. Jupiter’s magnetic field captures and literally slings charged particles from the Sun, creating the solar system’s most spectacular, and deadly, radiation belt. Landing on the surface and sticking your head out the airlock door while wearing an ordinary, unshielded astronaut’s helmet would be indistinguishable from sticking your face into an uncertified atomic accelerator. A crewed Europa probe would have to carry magnetic shield deflectors and other hardeners against radiation, and it would also have to be capable of penetrating tectonically active ice, then returning safely to the surface.
This may not be as easy as it sounds. A major consideration in the design of spacecraft has always been fuel economy. Yet dolphins need water, lots of water, simply for exercise. You cannot merely keep a dolphin in a bathtub during a three-year voyage (or three months, with a Valkyrie Mark II nuclear engine), and expect her to love you and go out into the great unknown and risk her life for you when you get there. This means (until or unless a more efficient means of Earth-to-orbit flight is evolved) at least a dozen of today’s shuttle flights just to carry seawater into space. Add to this, the tens of metric tons of extra fuel needed to nudge that extra mass of water out of Earth orbit and toward Jupiter, and the fuel that is needed to push that extra mass of fuel. Even with the most efficient fission-powered engines now available, including Franklin Chang-Diaz’s Vasmir (an ion-propelled predecessor to Valkyrie), the ship’s expanding dimensions become a significant problem in their own right.
It will not be much easier to send, say, a skeleton crew of four humans who will require living space, bulky life-support equipment, and rooms full of food to sustain them for (given only primitive, near-term propulsion systems) several years. “Weight” and fuel considerations are so overmastering that the ability to send men and/or dolphins beyond the orbit of Mars will not become a reality until the building of faster ships with very strong fuels contained in very small volumes (as, for example, anti-hydrogen as a fusion trigger) becomes practical. [1] Until then, we will have to satisfy ourselves with robot probes: land rovers that can locate thin ice and send down robot submarines cradled on nuclear reactors cooled by melting ice (as proposed in 1884’s Powell-Pellegrino design).
If launching robot submarines into space sounds a bit odd (though perhaps not as imaginative as dolphins) – well, why not robot helicopters for Titan? The idea brought forth a chorus of chuckles, in 1981, when I mentioned it in a Wellington tea room full of zoologists. A year later, as relations between New Zealand and the United States began to sour and as self-styled “scientific creationists” (Read: religious extremists) began to gain more than the “fair and balanced” share of the “equal time” they had been pleading for all along, I discovered that I could not possibly have picked a worse year to be talking about paleo-DNA and space probes, or to be publishing a book titled Darwin’s Universe. When I first arrived in New Zealand, it was a largely unexplored biological wonder-world with natural beauty unmatched anywhere else on Earth. On the week I left, in May of 1982, my two laboratories were being ransacked, someone had spray-painted “U.S. OUT! USSR STAY OUT!” on my home, and an upside-down car was burning in the street. Paradise had discovered the snake. (Oh, well – time to move on). Immediately before my departure, when I was called before what was evolving quickly into the “Ad-hoc Tribunal,” my funny tearoom discussion, a year earlier, was recited for me in vivid detail. Evidently, I had irritated people, and evidently these people had never heard of Kerry T. Nock. One of the tribunal participants commented, “These helicopters and submarines of yours – in space?” (smirking indulgently) “Won’t they fall down?”
It’s easy to turn such machines into a cartoons, and then criticize them because they look like cartoons. No good marksmanship required: a cartoonist need only fit the arrows into the holes he makes for them. (Even in the 21st century, if a President should start talking about Mars or Europa, he or she becomes an easy target for laughter, and for accusations of “being way out there – out past Pluto.”) While perhaps too imaginative, to a few, space-probe helicopters and submarines are not so crazy as they might seem at first hearing. Titan, for example, with a favorable combination of low gravity and dense atmosphere, is one of the most accessible bodies in the solar system for exploration by robot spacecraft. A Titan heliprobe could enter the atmosphere in a protective shell, or capsule, then land in much the same manner as the Mars rovers. Powered by batteries (charged by a small fission reactor) and possessing stubbier rotar blades than terrestrial helicopters, the robot could perform a gadabout exploration of the world, wheeling about in one place for weeks or even months, then lifting off to explore what lies beyond the next mountain pass. Simplifying the operation, once more is known about Titan’s surface, it may be possible to reduce the reactor in both mass and size, to a backup system for a gas-electric hybrid engine. Hydrocarbons drawn from the atmosphere and from stream-beds could be combined with oxygen drawn from rocks (which appear to be composed mostly of ice, as rocks on Earth’s surface are composed mostly of silicon).
Titan buoyant stations, proposed by the Jet Propulsion Laboratory Advanced Projects Group, have also been designed and considered. Group supervisor Kerry Nock explains: “We were studying both hot air and gas (H2) filled balloons. Some concepts, which carry electric engines to power propellers, look more like the Goodyear blimp than a balloon. Surface samplers could be lowered from and raised to the stations on tethers.” At Titan’s most tantalizing locations, some of them worth weeks of scrutiny, the entire “gondola” might descend, spider-like, on a tether, and take anchor for a while.
Looking outward from our Earth, and using our imaginations as we peer down through the skin of a Saturnian moon, we are awakened to the dawning reality that Charles Darwin’s “warm little pond” – the pools and rivers of a terrestrial globe like Earth – must not be a universal and invariable requirement for the origin of life.
It can happen almost anywhere, given the galactic wild card of icy desert worlds with their life underground.
It can happen near every star in the sky.
During the Gulf War and all of the other prominent, difficult news of the past three years, the discovery that Mars is essentially Europa with most of its skin stripped off, and revelations of ancient Martian seas that lasted for millions of years (probably a half billion years, or more) and produced hematite microspheres of the sort matched on Earth only by deep-ocean bacterial Consorms in the presence of hydrothermal vents, has been entirely missed by the public. Aesthetically stunning photos are coming down from Mars and Saturn every day or two – and most people have never seen them. Even my children (who have heard too much about Mars and Titan), respond with genuine Oohs and ahhs when seeing these other-worldly postcards and 3-D panoramas for the first time. And the best is yet to come. In January, the Cassini/Huygens lander will descend to the surface of Titan. A month later, Cassini will make its first close fly-by of Enceladus. The results of the Galileo mission are just now being summarized, following a decision by NASA (knowing that Galileo was an unsterilized spacecraft and that Earthly bacteria on the moon had survived inside the Surveyor lander’s camera, until brought back to Earth by the crew of Apollo 12, years later), to intentionally crash Galileo into Jupiter’s cloud-tops, and burn it to atoms, so as not to risk the robot crashing through Europa’s ice and contaminating whatever life may dwell there, with bacteria from Earth. New oceans are turning up throughout the solar system – and to a near certainty –life, of at least the microbial variety.
These are indeed interesting times. For reasons worthy of their own chapter, it has turned out (according to a theory written by me and Francis Crick, about the time-curve of heavy element accumulation rates in the galaxy) – that if we fail to find worlds like Europa and Enceladus and Titan to be capable of generating anything more advanced than bacteria, we, on Earth, may find ourselves to be among the only – if not the only, advanced multicellular life forms in the galaxy. According to the theory of “Genesis and Galactic Blight,” if seas in ice-worlds are not truly a biological wild card, we may truly be all there is.
As a child, one of my greatest heroes was William Beebe, who went down nearly three hundred meters, all alone, in a one-man bathysphere. I’ve always been impressed with the people who went alone – John Glen in his Mercury capsule, Mike Collins orbiting alone above the moon while Neil and Buzz were on the surface. I’ve been awed by them, and I’ve envied them. William Beebe’s dive was barely a training dive for us today; and he kept meticulous notes, and he told Charles Lindbergh that he was scared to death. In Beebee’s tradition, I too kept meticulous notes of the changes seen in the ocean, during every ten meter interval of our trip to the bottom. On every dive, I was the only person whose face was glued to the plexiglass, every minute, down and up. And always my mind was with Beebe. It’s more wonderful than Earth orbit down there and, I think, perhaps even more beautiful, though it is impossible to be sitting near a volcanic vent surrounded by a deep-ocean zoo filled with creatures no one has ever seen before, and not to be thinking about Europa – impossible not to be down there on the bed of the ocean, and glancing always up toward space. I do not believe any book has ever described, in any detail, the sheer magic of one of these dives. No one in the world (not even an astronaut) has such a visually stunning commute to work. Given a choice to go back down there, or into earth orbit, I’d go back down there even though I’ve already been there several times before.
There is also something to be said for the serendipitous nature of scientific discovery. While down there, testing our machines in a “dry-run” for Europa, we found a creature that, aside from coming close to being the common ancestor of us all, began to teach us how the immune system was probably evolved – a discovery that is, as I write, pointing the way toward a possible new anti-cancer agent that may work “without the cure becoming as sickening as the disease.” The serendipitous nature of scientific discovery should never be disregarded. We would never have found this “deep-sea alien” if our eyes had not been pointed toward Europa. Often enough in science, either a cure for a disease or the door to a new frontier is discovered by accident while looking for something else.
Here, aboard Keldysh and in the Mirs and Deep Rovers, for the first time in history, the once separate worlds of space exploration and deep-ocean exploration have become intimately entwined. Strangely, and perhaps predictably, these expeditions are being carried out jointly by formerly adversarial Russian and American crews, who have during the past three years become family (with one inter-crew marriage already on record; this, in accordance with a former colleague of Dr. Keldysh, who in 1991, during the U.S. Soviet Space Cooperation Initiative, and at the start of the first joint oceanographic expedition, said, “I have a dream that one day, your children and my children will play together”).
So there is, at bottom, a very human history behind these explorations. With Cassini now safely within Saturn orbit (my colleagues and I waited for 20 years, biting our nails this spring to learn whether or not, from 1.5 light hours away, we would receive “word” that the braking rocket had fired unfailingly) – the coming year promises to be the most interesting in the entire history of deep-space and deep-ocean exploration. (Already, we have found the only precise, Earthly analog of the Mars hematite concretions known as “blueberries” in a bacterial reef discovered at a deep-ocean volcanic vent – discovered only this August past). Most of this book will deal, in a you-are-there manner, with these new worlds – above and below – and they will be written about in the style of Chapter 1, above.
One of my aims, of course, is to communicate an exciting future to tomorrow’s explorers – to drive home the message that there are vast and fantastic new frontiers to explore, and that there is a future worth having, if our civilization is wise, and pays attention. (As Ghosts of Vesuvius was a book looking to the past and unearthing signposts to the future, this book dwells in the opposite direction, in the future indicated in GOV). This book will not be entirely about science, however. Science is, in its deepest essentials a human adventure, with human moments. We are, after all – we of the Keldysh and the Mirs – carrying out the oceanographic half of these voyages, and the testing of spacefaring robots – aboard a ship named after the scientist who had dreamed (when he first proposed a joint Russian-American adventure into the darkest and most dangerous places): That by learning to survive and work together in the cold and the dark – in space as a prelude to Mars and in the deeps as a prelude to Europa – that adversarial nations might discover, all alone in the night, their common humanity.
Today, the dream is alive.
[1] At Brookhaven National Laboratory, beginning in 1984, James Powell, Pierre Noyes, Charles Pellegrino and Hiroshi Takahashi designed such ships. Accelerator-assisted antimatter-triggered fusion propulsion appears feasible within two or three decades. Indeed, serendipitous advances made in 2004 rendered the efficiency of anti-proton production (stabilized as super-cooled, magnetically confined anti-hydrogen) some 10,000 times more efficient than only a short time before had been believed possible given even a half century of new enabling technology. The more complex Valkyrie Mark III brings interstellar distances within human reach, at maximum cruising velocities of 0.92c. The Valkyrie Mark II can easily accelerate large, crewed spacecraft up to 360 kilometers (220 miles) per second, bringing Jupiter within only a few months’ distance. Antimatter engine concept tests (using only sub-microscopic amounts of material) may begin as early as 2010. However, the weapons potential of heavy ions and even ice crystals accelerated to relativistic velocity (the r-bomb, or relativistic high-mass collider) arising from antimatter technology is every bit as bone-chilling as the Cold War’s “balance of terror.” And Pellegrino asks, “Have we already created something we cannot give back?” As a jokingly delivered reply – and perhaps not so jokingly – a Shinnecock tribal elder has given Pellegrino a passage from Nostradamus, asking whether or not it might refer to antimatter: “The contrary of the positive ray shall exterminate all.”
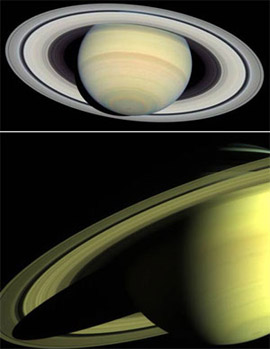
Saturn